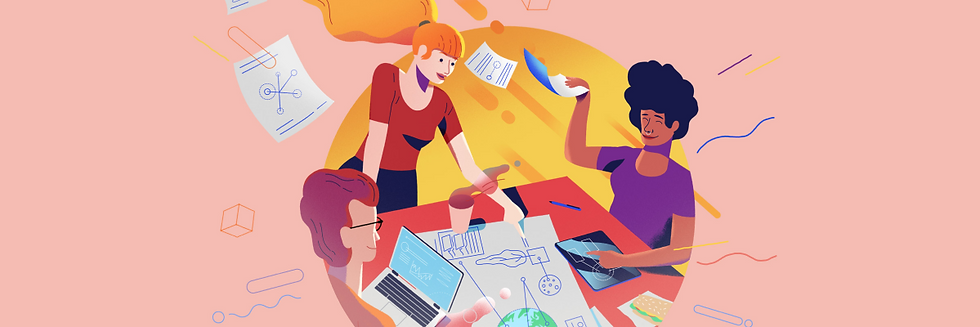
Benefits of joining the SPARKLE network
Competitive salary
The Marie-Curie Action guarantees competitive salary which accounts for the institution, family situation and the country.
European-wide training
The network offer state-of-the-art training in solid state physics and optics, both experimental and theoretical. You will have to the opportunity to travel across Europe for our planned workshops, schools, code hackathons, and conferences in leading Universities and Research Institutions.
Extended External Stays across Academia and Industry
All the PhD positions include funded research stays in the network nodes of beneficiaries and associated partners which include leading companies in material and light technologies.
THEORY
The Role of Coherence in Light-Matter Photogroundstates
Supervisor:
Prof. Simone Latini
Description: Can vacuum photon fluctuations—tiny quantum “wiggles” of the electromagnetic field—actually reshape a material’s ground state? This Ph.D. project focuses on extracting new insight from measurable light signals in systems where photons and matter strongly interact. By integrating Maxwell’s equations with density functional theory, we will identify which photonic and material parameters enable conditions such as exciton-polariton condensation. We will then use Green’s function methods to see how matter correlations become “written” into photonic observables—revealing quantum phases through the light they emit or transmit. Emphasis will be placed on bilayer 2D materials with tunable excitons, offering a rich platform for studying how coherence in light can serve as a window into exotic quantum states.
Learning Outcomes: You will develop expertise in computational photonics, quantum optics, and many-body theory, alongside hands-on experience with advanced simulation tools and high-performance computing. These skills will prepare you for a future at the cutting edge of quantum materials research, whether in academia or industry.
THEORY
Control of Composite Particles of Hybrid Light–Matter Nature
Supervisor:
Prof. Simone Latini
Description: This Ph.D. project explores how real photons inside optical cavities can drive stronger light–matter interactions and create new quantum phases. Using a combination of Quantum Electrodynamics (QED) and Non-Equilibrium Green’s Function (NEGF) methods, we will investigate composite particles that go beyond simple exciton–photon pairs—incorporating more than two quasiparticles at once. Through simulations, we aim to precisely control how cavities are driven, enabling ultrafast “mixing” and “un-mixing” of polaritons. This approach will help us manipulate symmetry-breaking phenomena in real time, ultimately leading to exotic states that blend classical light, quantum light, and excitons. Our work promises insights into all-optical control of materials, with a focus on tuning confinement and adding real photons to achieve robust hybrid phases and unique absorption properties.
Learning Outcomes: You will acquire hands-on expertise in computational physics and quantum optics, with a focus on QED and NEGF. High-performance computing and advanced simulation techniques will sharpen your programming and modeling skills. This project will prepare you for frontier research in quantum technologies, bridging academia and next-generation industrial applications.
Official call: link
For further info on our research visit here
THEORY
Computational Floquet Band Engineering in Solids with Tailored Lasers
Supervisor:
Dr. Hannes Hübener
Description: Floquet band engineering offers a powerful way to dynamically reshape a solid’s electronic properties using periodic light fields, opening new frontiers in ultrafast optoelectronics and quantum materials. This Ph.D. project advances Time-Dependent Density Functional Theory (TDDFT) to explore how tailored lasers can reconfigure electronic band structures and induce novel states of matter. By integrating Floquet theory with TDDFT, we will investigate high-harmonic generation, light-induced phase transitions, and optimize laser parameters for precise material manipulation.
The project involves developing computational tools to simulate laser-driven dynamics in solids, focusing on combining multiple laser frequencies to achieve targeted band engineering. Applications to materials like graphene and transition metal dichalcogenides will highlight how customized laser fields can create exotic phases, including topologically protected states or flat bands. High-performance computing will enable the study of these complex systems over extended timescales.
Learning Outcomes: You will gain expertise in computational physics and advanced electronic-structure methods, particularly TDDFT and Floquet theory. Practical skills in programming, high-performance computing, and numerical modeling of ultrafast light–matter interactions will be essential. This experience will equip you for cutting-edge research in academia, quantum technology, or advanced materials industries.
Official call: link
For further info on our research visit here
THEORY
Tr-ARPES of Light-Dressed Surface States
Supervisor:
Prof. Umberto de Giovannini
Description: This Ph.D. project focuses on developing advanced theoretical tools to simulate time-resolved angle-resolved photoemission (Tr-ARPES) and uncover how intense lasers can alter surface electronic states. We will investigate a wide range of materials—from 2D systems like graphene and bismuthane (in ribbon forms) to 3D topological insulators (e.g., Na3Bi) and molecular films on metals. By combining first-principles approaches (such as TDDFT) with ab initio tight-binding models, we aim to clarify how “Floquet-dressing” of surface states arises under strong laser driving and multi-color excitation schemes. Photoemission orbital tomography will serve as a key diagnostic tool, revealing how topological properties and molecular states evolve in real time. These insights will open up new ways to manipulate surface states, paving the way toward innovative optoelectronic and spintronic applications.
Learning Outcomes: You will gain hands-on experience with cutting-edge computational physics methods, including TDDFT, tight-binding models, and high-performance computing. By tackling the simulation of surface phenomena and time-resolved spectroscopy, you will develop strong programming skills and a deep understanding of how lasers can induce and control topological effects. This expertise will prepare you for advanced research roles in academia or industry, focusing on quantum materials and ultrafast science.
THEORY
Time-resolved spectroscopy with quantum light
Supervisor:
Prof. Umberto de Giovannini
Description: This Ph.D. project aims to develop an ab initio theoretical framework for studying time-resolved photoemission (ARPES) and absorption/reflection spectroscopies in systems strongly coupled to quantum light. Building on a newly introduced photon-free functional from quantum-electrodynamical density-functional theory (QED-DFT), we will extend this functional—originally formulated in equilibrium—to include explicit time dependence. This extension will then be combined with computational techniques such as tSURFF for ARPES and advanced methods for time-resolved absorption spectroscopy. By exploring non-perturbative regimes, such as high-harmonic generation (HHG), we expect to gain deeper insights into how quantum light influences electronic excitations and material properties.
Learning Outcomes: You will develop strong skills in computational physics, including density-functional theory, quantum optics, and many-body methods. This project also offers hands-on experience in time-resolved spectroscopy, enabling you to bridge theory and experiment. Through collaborations with experimental groups, you will learn to align theoretical predictions with real-world measurements, preparing you for a cutting-edge career in quantum materials research.
Official call: link
For further info on our research visit here
THEORY
Charge transport under vibrational strong and ultrastrong coupling
Supervisor:
Prof. Guido Pupillo & Dr. David Hagenmuller
Description: In several organic semiconductors, vibrational modes can play a surprisingly big role in how charges move through the material. This Ph.D. project explores whether we can tune charge conductivity by coupling these vibrational transitions to the electromagnetic field inside an optical cavity—a regime often called “vibrational strong” or even “ultrastrong” coupling. We will investigate different material systems and cavity geometries to see how light–matter interactions can modify charge transport, with potential applications in optoelectronics and photovoltaics.
Learning Outcomes: You will gain hands-on experience in computational physics and quantum optics, learning how to model and interpret strong light–matter coupling scenarios. By the end of this project, you will be proficient in simulating charge transport and designing cavities for advanced photonic materials. This skill set opens up opportunities across academia and industry, especially in developing new strategies for energy harvesting and electronic devices.
Official call: link
For further info on our research visit here
THEORY
Description: This Ph.D. project investigates how excitons interact with, and even reshape, magnetic order in Mott and charge-transfer insulators. First, we will explore how excitons form and evolve within these strongly correlated environments, highlighting their impact on the magnetic structures. Next, we will study the emergence of exciton-polaritons—hybrid states formed when excitons couple strongly to light—to see how external illumination can modify magnetic properties. To accomplish these goals, we will develop a novel approach based on Dynamical Mean-Field Theory (DMFT), enhanced by a two-particle self-consistency framework for light–matter interactions. Finally, by incorporating ab initio-derived parameters for materials like NiPS₃ and NiO, we will ensure that our theoretical predictions closely match experimental conditions.
Learning Outcomes: You will gain expertise in theoretical condensed matter physics, particularly DMFT and two-particle response theory, as well as computational techniques for modeling strongly correlated materials. This training will position you at the forefront of research bridging theory, simulation, and experiment, preparing you for a career in academia or high-tech industries focused on emerging quantum materials.
Official call: link
For further info on our research visit here
Excitonic Interactions and Light-Matter Coupling in Strongly Correlated Systems
Supervisor:
Prof. Denis Golež
THEORY
Random Lasers Based on Perovskite Materials
Supervisor:
Dr. Sol Carretero Palacios
Description: This Ph.D. project aims to develop and fabricate perovskite-based random lasers, combining both theoretical and experimental approaches. On the theoretical side, you will create a Monte Carlo model to predict light scattering and lasing in these systems, with a focus on optimizing device performance. In parallel, you will synthesize and characterize perovskite materials to validate your simulations and gain insight into how structural inhomogeneities affect lasing efficiency and stability. By bridging simulation and experiment, you will uncover strategies to enhance the performance of perovskite random lasers, paving the way for novel photonic applications.
Learning Outcomes: You will gain experience in computational modeling (Monte Carlo simulations), materials synthesis (perovskite fabrication), and optical characterization (laser performance measurements). This balanced training in both theory and experiments will prepare you for cutting-edge research in photonics, materials science, and beyond, building a strong foundation for a career in academia or high-tech industry.
Official call: link
For further info on our research visit here
EXPERIMENT
Cavity-Mediated Vibrational Control of the Metal–Insulator Transition in Ca₂RuO₄
Supervisor:
Prof. Daniele Fausti
Description: This Ph.D. project aims to investigate how optical cavities can be used to tune and control the metal–insulator transition in Ca₂RuO₄, a multiband Mott system. By placing the material inside a resonant cavity, we plan to modify vibrational modes—specifically infrared-active phonons—so that they couple more strongly with other degrees of freedom. Through techniques like broadband optical spectroscopy, time-resolved Raman, and resonant inelastic x-ray scattering, we will see how cavity hybridization affects the material’s equilibrium properties. We will then drive these modified vibrational modes with ultrashort mid-IR pulses that match the cavity’s resonant frequencies. The goal is to achieve non-linear “phononic” control of the phase transition, allowing us to switch Ca₂RuO₄ between metallic and insulating states by tailoring Rabi splitting and excitation parameters. Ultimately, we seek to identify the optimal conditions for dynamically steering this material’s electronic phase, opening pathways toward advanced photonic and quantum devices.
Learning Outcomes: You will gain hands-on expertise in ultrafast spectroscopy, photonic cavity design, and materials characterization. By working across disciplines—combining experimental optics, condensed matter physics, and advanced data analysis—you will develop a versatile skill set applicable to both fundamental research and emerging technologies in light-driven quantum control.
Official call: link
For further info on our research visit here
EXPERIMENT
Engineering Topological Phases in Light-Driven Quantum Materials
Supervisor:
Dr. Samuel Beaulieu
Description: We are seeking a motivated Ph.D. student to investigate how ultrafast laser pulses can break specific symmetries in quantum materials and unlock novel topological phases. Symmetry is central to defining electronic properties in condensed matter, and dynamically controlling these symmetries with intense, tailored laser fields offers a path toward discovering entirely new states. You will employ cutting-edge techniques—such as time-, angle-, and polarization-resolved photoemission spectroscopy—to capture and analyze these emergent properties in real time.
Hosted at the Centre Lasers Intenses et Applications (CELIA) in Bordeaux, this project provides access to state-of-the-art ultrafast laser facilities and a stimulating research environment. The city of Bordeaux, renowned for its cultural heritage and vibrant community, offers an ideal setting for both scientific and personal growth.
Learning Outcomes: You will gain expertise in ultrafast spectroscopy, advanced photoemission techniques, and the physics of quantum materials. By manipulating and measuring topological phases under dynamic conditions, you will develop skills that are highly valuable in both academic research and the broader field of photonic and quantum technologies.
Official call: link
For further info on our research visit here
EXPERIMENT
Non-perturbative light-matter interaction in solids
Supervisor:
Dr. Caterina Vozzi
Description: This Ph.D. project at CNR aims to push the limits of ultrafast spectroscopy in 2D Metal Halide Perovskites by combining intense terahertz (THz) pulses with high-harmonic generation and time-resolved X-ray absorption techniques. Our goal is to understand how excitons and phonons interact under extreme non-perturbative conditions, focusing on the formation and dissociation of polaron–excitons. Using state-of-the-art facilities at the UDynI labs (www.udyni.eu), you will investigate how these interactions shape the optoelectronic properties of perovskites, paving the way for innovative device applications.
Learning Outcomes:
By working on advanced laser systems, THz pumping, and X-ray absorption setups, you will gain hands-on experience in designing and conducting ultrafast experiments. You will learn sophisticated data analysis and interpretation techniques, honing your ability to tackle complex questions in condensed matter physics. This project provides a strong foundation for a future career in ultrafast science, photonics, or materials research, bridging fundamental discoveries and real-world applications.Official call: link
For further info on our research visit (here)
EXPERIMENT
Polaritonic driving of bilayer graphene and transient devices
Supervisor:
Prof. Hanan Herzig Sheinfux
Description: This Ph.D. project explores the frontiers of light–matter interaction by exploiting polaritonic effects in bilayer graphene. While periodically driving electrons with lasers can reshape electronic band structures, we aim to push these boundaries by forcing the laser field to vary at the nanoscale. This approach promises unique physics and could enable robust control of bilayer graphene’s electronic properties, paving the way for new types of optoelectronic devices.
In addition to fundamental research on how 2D materials interact with precisely tuned laser fields, we will examine transient device applications. By studying ultrafast processes and understanding how polaritonic coupling alters material behavior, the project seeks to lay the groundwork for innovative photonic technologies.
Learning Outcomes: You will gain expertise in ultrafast laser techniques, polaritonic physics, and advanced simulation or experimental methods for probing band structures. This broad skill set will prepare you for cutting-edge research and technology development, positioning you at the intersection of nanoscience, optics, and device engineering.
Official call: link
For further info on our research visit here
EXPERIMENT
Ultrafast Magnetometry of Floquet-Engineered Systems
Supervisor:
Prof. Gregor Jotzu
Description: This Ph.D. project focuses on developing ultrafast magnetometry tools to probe periodically driven solids on the sub-picosecond timescale. When materials are excited with intense mid-infrared or terahertz light, they can enter “Floquet-engineered” phases that exhibit novel properties—often with no static equivalent. However, these phases only persist while the drive is active, making ultrafast and spatially resolved measurement techniques essential. By refining magnetometric probes to distinguish between bulk and edge responses, we aim to explore topologically non-trivial edge states that show unique magnetic signatures.
Learning Outcomes: You will gain expertise in ultrafast optics, magnetometry, and topological materials research. Through hands-on experience in advanced spectroscopic methods and high-speed data acquisition, you will develop the skills needed to investigate and control complex phases of matter, preparing you for cutting-edge roles in academic and industrial research.
Official call: link
For further info on our research visit here
EXPERIMENT
Integrated photonics for studies of quantum electrodynamics in coupled light-matter systems
Supervisor:
Prof. Ileana-Cristina Benea-Chelmus
Description: Cavity-confined electromagnetic fields can establish correlations among otherwise unconnected quantum systems, especially in the terahertz (THz) range—where excitations like Landau levels, interband transitions, and roto-vibrational modes are abundant. Yet, directly observing these fields and their correlations remains a challenge. Recent advances in integrated photonics allow sub-cycle spatiotemporal sampling of THz waves on-chip, routing optical probes through carefully designed waveguides into highly confining THz elements. These structures not only boost the vacuum field and any resulting light–matter coupling but also define and limit the precise region in which the interaction occurs.
This Ph.D. project aims to leverage these emerging techniques for on-chip field correlation measurements, with the goals of: (i) Investigating the quantum optical properties of THz fields in integrated circuits, (ii) Exploring the role of long-range correlations in coupled light–matter systems via hybrid integration (iii) Developing methods for quantum-level detection and read-out within photonic platforms.
Learning Outcomes: You will gain expertise in integrated photonic circuit design and fabrication, high-precision THz spectroscopy, and advanced quantum optical measurement strategies. This skill set will prepare you for cutting-edge research and development in quantum photonics, materials science, or related high-tech industries.
Official call: link
For further info on our research visit here
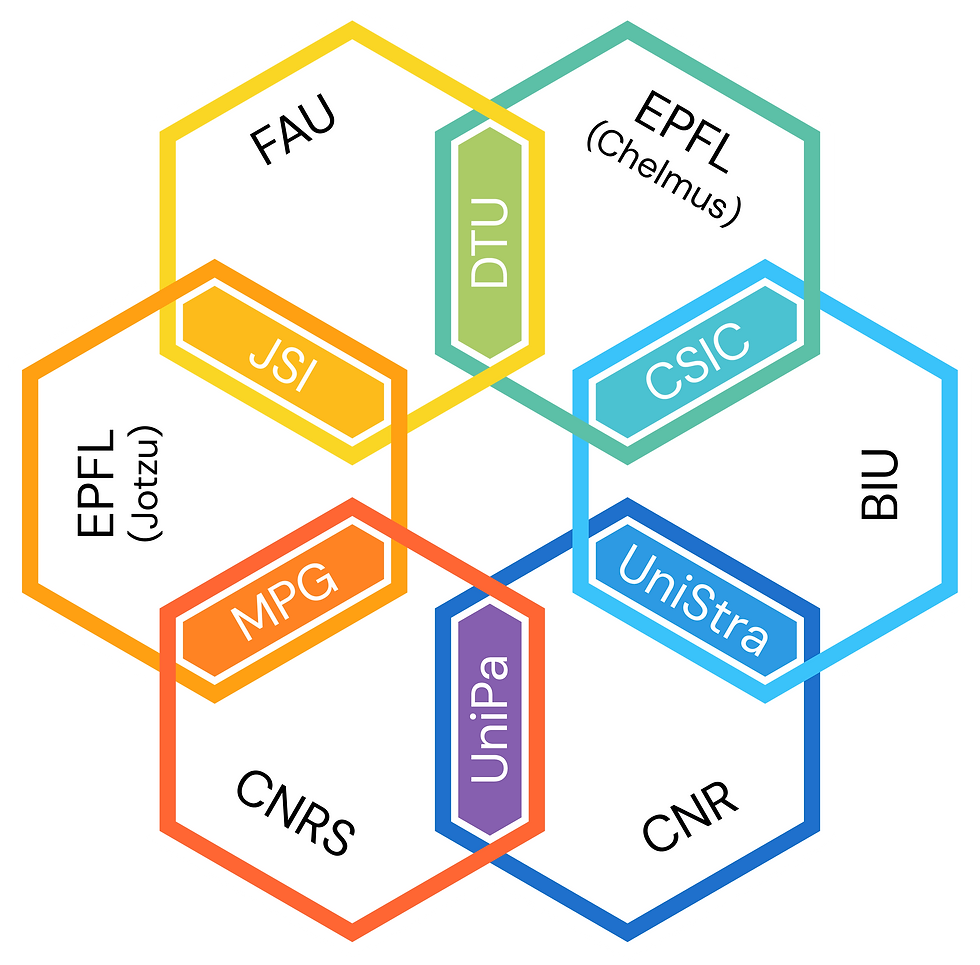
You will become an independent well-round researcher with an attractive profile for both Academia and Industry!
Benefits of joining the SPARKLE network
Competitive salary
The Marie-Curie Action guarantees competitive salary which accounts for the institution, family situation and the country.
European-wide training
The network offer state-of-the-art training in solid state physics and optics, both experimental and theoretical. You will have to the opportunity to travel across Europe for our planned workshops, schools, code hackathons, and conferences in leading Universities and Research Institutions.
Extended External Stays across Academia and Industry
All the PhD positions include funded research stays in the network nodes of beneficiaries and associated partners which include leading companies in material and light technologies.
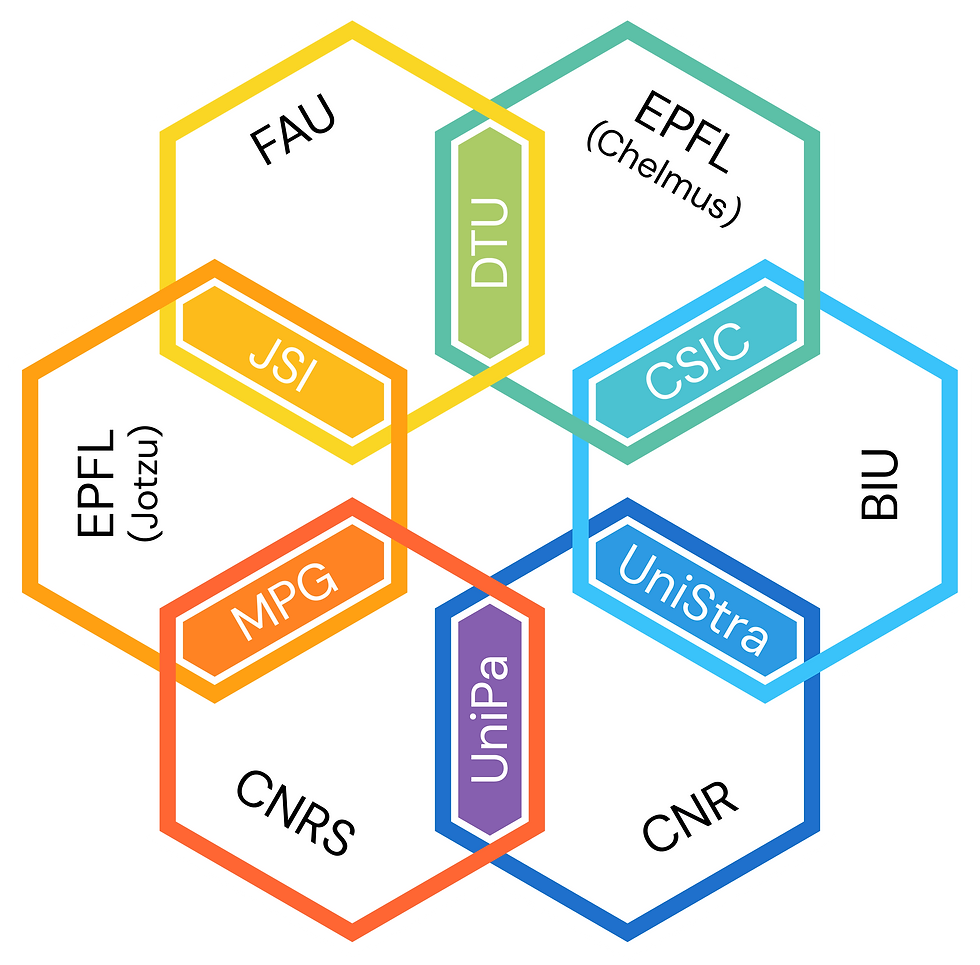